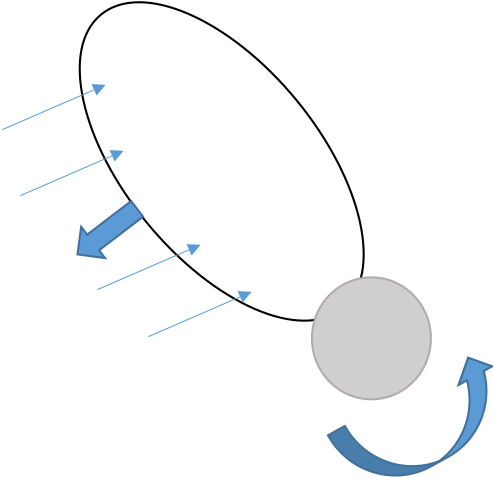
Published 2021-03-22
Keywords
- Cavitation,
- degassing,
- hollow fibre membrane,
- propeller
How to Cite
Copyright (c) 2020 Mojtaba Taseidifar , John Antony, Richard Pashley

This work is licensed under a Creative Commons Attribution 4.0 International License.
Abstract
It is well known that cavitation phenomena affect the efficiency of propellers. It is a major world wide economic problem for the transport industry. The speed of fast, ocean going vessels is liimted by cavitation effects on hydrofoils and propulsion systems. The main approaches of industry to mitigate the detrimental effects of cavitation on propellers is restricted to varying operating conditions, geometric design and choice of wear resistant materials. We here develop a simple solution to the problem. It has been known for over a century that dissolved gases reduce the tensile strength of liquids by orders of magnitude. De-gassing of a iquid dramatically reduces the ability of a fluid to cavitate. Propeller cavitation in ships and submarines is typically controlled by reducing rotation rate and/or blade pitch. We here demonstrate the astonishing fact that cavitation can be completely prevented by releasing de-gassed water adjacent to the low pressure side of a rotating propeller, without varying blade speed or pitch. Practical implementation is simple and cheap.
References
- H. Kato, Cavitation, in Advances in Marine hydrodynamics, Computational mechanics publications, 1996.
- C.E. Brennen, Cavitation and Bubble Dynamics, Oxford University Press, 1995.
- L. Rayleigh, VIII. On the pressure developed in a liquid during the collapse of a spherical cavity, The London, Edinburgh, and Dublin Philosophical Magazine and Journal of Science, 1917, 34 (200), 94-98.
- R. M. Pashley, P. M. McGuiggan, B. W. Ninham, D. F. Evans, Attractive forces between uncharged hydrophobic surfaces: direct measurements in aqueous solution, Sci., 1985, 229(4718), 1088-1089.
- R. W. Johnson, Handbook of Fluid Dynamics, 1998, CRC Press.
- Z. Pan, A. Kiyama, Y. Tagawa, D. J. Daily, S. L. Thomson, R. Hurd, T. T. Truscott, Cavitation onset caused by acceleration, Proc. Nat. Acad. Sci., 2017, 114(32), 8470-8474.
- J. N. Israelachvili, R. M. Pashley, The hydrophobic interaction is long range, decaying exponentially with distance, Nature, 1982, 300(5890), 341-342.
- H. K. Christenson, J. N. lsraelachvili, R. M. Pashloy, Properties of Capillary Fluids at the Microscopic Level, SPE Res. Eng., 1987, 155-165.
- M. Alheshibri, J. Qian, M. Jehannin, V.S.J. Craig, A history of nanobubbles, Langmuir, 2016, 32, 11086?11100.
- A. Agarwal, W. J. Ng, Y. Liu, Principle and applications of microbubble and nanobubble technology for water treatment, Chemosphere, 2011, 84, 1175-1180.
- Z. Fang, X. Wang, L. Zhou, L. Zhang, J. Hu, Formation and Stability of Bulk Nanobubbles by Vibration, Langmuir, 2020, 36, 2264?2270.
- R. M. Pashley, Effect of degassing on the formation and stability of surfactant-free emulsions and fine teflon dispersions, J. Phys. Chem. B, 2003, 107(7), 1714-1720.
- M. Rzechowicz, R. M. Pashley, A membrane method for degassing nonaqueous liquids, J. Colloid Interface Sci., 2006, 298(1), 321-326.
- M. Rzechowicz, R. M. Pashley, The effect of de-gassing on the efficiency of reverse osmosis filtration, J. Membr. Sci., 2007, 295(1), 102-107.
- M. Ashokkumar, The characterization of acoustic cavitation bubbles – An overview. Ultrason. Sonochem., 2011, 18(4), 864-872.
- J. Frohly, S. Labouret, C. Bruneel, I. Looten-Baquet, R. Torguet, Ultrasonic cavitation monitoring by acoustic noise power measurement, J. Acoust. Soc. Am., 2000, 108(5) 2012-2020.
- Q. Zheng, D.J. Durben, G.H. Wolf, C.A. Angell, Liquids at Large Negative Pressures: Water at the Homogeneous Nucleation Limit, Sci., 1991, 254(5033), 829-832.
- W.J. Galloway, An Experimental Study of Acoustically Induced Cavitation in Liquids, J. Acoust. Soc. Am., 1954, 26(5) 849-857.
- M.J. Francis, R.M. Pashley, M. Rzechowicz, The effects of feed water de-gassing on the permeate flux of a small scale SWRO pilot plant. Desalination and Water Treat., 2011, 25(1-3) 150-158.
- J.H. Arakeri, P.N. Shankar, Ludwig Prandtl and boundary layers in fluid flow. Resonance, 2000, 5(12) 48-63.
- H. Schlichting, K. Gersten, Fundamentals of Boundary–Layer Theory, in Boundary-Layer Theory, Springer Berlin Heidelberg: Berlin, Heidelberg, 2017, 29-49.
- R. M. Pashley, M. Rzechowicz, L. R. Pahley, M. J. Francis, De-Gassed Water Is a Better Cleaning Agent, J. Phys. Chem. B, 2005, 109(3) 1231-1238.