Light-Modulated Rheological Properties in Green Innovative Formulations
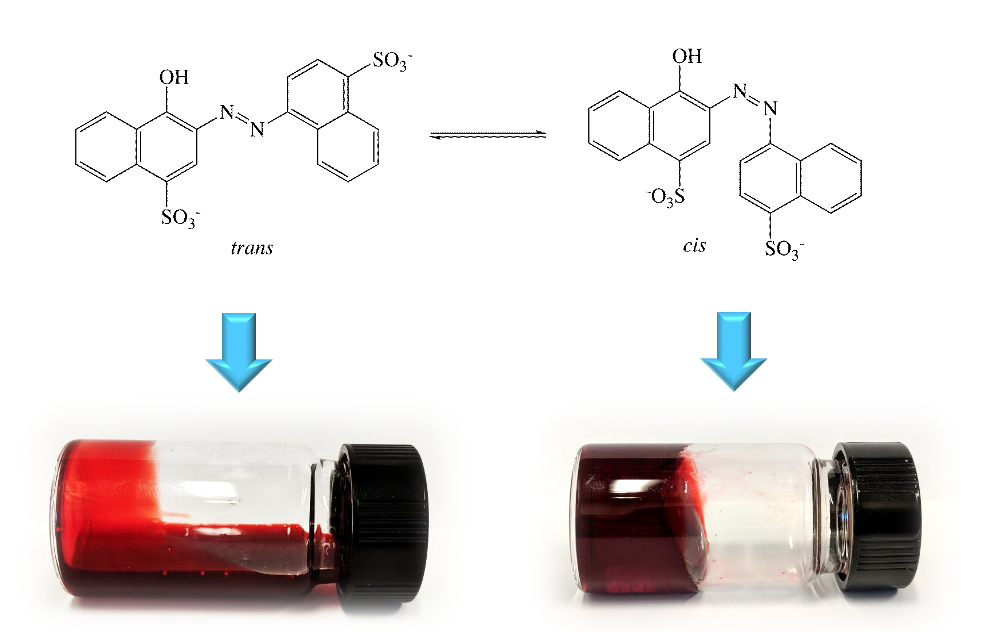
Published 2022-09-01
Keywords
- green chemistry,
- sodium oleate,
- azorubine,
- viscosity,
- stimulus-responsive
How to Cite
Copyright (c) 2022 Duccio Tatini, Martina Raudino, Filippo Sarri

This work is licensed under a Creative Commons Attribution 4.0 International License.
Abstract
The addition of azorubine to a viscoelastic aqueous dispersion of sodium oleate (NaOL, 0.43 M, 13% w/w) and KCl (up to 4% w/w) leads to a green gel-like system whose rheological behavior can be efficiently and reversibly triggered from remote by using UV light. Rheology, Differential Scanning Calorimetry (DSC) measurements and phase behavior studies indicate that the original texture of the NaOL dispersion is significantly hardened upon UV irradiation for 8 hours in the presence of azorubine, showing a seven hundred-fold increase in viscosity. The UV treatment brings about the trans to cis isomerization of azorubine, which modifies the structure of the NaOL wormlike micellar system, leading to a more entangled, close-textured network. The cooperative effect of KCl on the fluid viscosity is found to be concentration-dependent. The system slowly reverts to its original rheological behaviour after standing for about 1 day. These results are relevant for the development of stimuli-responsive innovative systems based on biocompatible, non expensive and commercially available materials that can be used in a wide range of applications, such as in drug delivery or enhanced oil recovery, where a quick change in the physico-chemical features of the system is required but difficult to be performed.
References
- M. Wei, Y. Gao, X. Li, M.J. Serpe, Stimuli-responsive polymers and their applications, Polym. Chem. 8 (2016) 127–143. https://doi.org/10.1039/C6PY01585A.
- C.A. Dreiss, Wormlike micelles: where do we stand? Recent developments, linear rheology and scattering techniques, Soft Matter. 3 (2007) 956–970. https://doi.org/10.1039/B705775J.
- S. Guragain, B.P. Bastakoti, V. Malgras, K. Nakashima, Y. Yamauchi, Multi-Stimuli-Responsive Polymeric Materials, Chem. Weinh. Bergstr. Ger. 21 (2015) 13164–13174. https://doi.org/10.1002/chem.201501101.
- E. Moulin, L. Faour, C.C. Carmona-Vargas, N. Giuseppone, From Molecular Machines to Stimuli-Responsive Materials, Adv. Mater. 32 (2020) 1906036. https://doi.org/10.1002/adma.201906036.
- S. Ahn, R.M. Kasi, S.-C. Kim, N. Sharma, Y. Zhou, Stimuli-responsive polymer gels, Soft Matter. 4 (2008) 1151–1157. https://doi.org/10.1039/B714376A.
- D. Wang, G. Wei, R. Dong, J. Hao, Multiresponsive Viscoelastic Vesicle Gels of Nonionic C12EO4 and Anionic AzoNa, Chem. – Eur. J. 19 (2013) 8253–8260. https://doi.org/10.1002/chem.201300132.
- M.A.C. Stuart, W.T.S. Huck, J. Genzer, M. Müller, C. Ober, M. Stamm, G.B. Sukhorukov, I. Szleifer, V.V. Tsukruk, M. Urban, F. Winnik, S. Zauscher, I. Luzinov, S. Minko, Emerging applications of stimuli-responsive polymer materials, Nat. Mater. 9 (2010) 101–113. https://doi.org/10.1038/nmat2614.
- J. Yang, Viscoelastic wormlike micelles and their applications, Curr. Opin. Colloid Interface Sci. 7 (2002) 276–281. https://doi.org/10.1016/S1359-0294(02)00071-7.
- H. Rehage, H. Hoffmann, Rheological properties of viscoelastic surfactant systems, J. Phys. Chem. 92 (1988) 4712–4719. https://doi.org/10.1021/j100327a031.
- H. Shi, Y. Wang, B. Fang, Y. Talmon, W. Ge, S.R. Raghavan, J.L. Zakin, Light-Responsive Threadlike Micelles as Drag Reducing Fluids with Enhanced Heat-Transfer Capabilities, Langmuir. 27 (2011) 5806–5813.
- M. Samuel, D. Polson, D. Graham, W. Kordziel, T. Waite, G. Waters, P.S. Vinod, D. Fu, R. Downey, Viscoelastic Surfactant Fracturing Fluids: Applications in Low Permeability Reservoirs, in: Society of Petroleum Engineers, 2000. https://doi.org/10.2118/60322-MS.
- C. Moitzi, N. Freiberger, O. Glatter, Viscoelastic Wormlike Micellar Solutions Made from Nonionic Surfactants: Structural Investigations by SANS and DLS, J. Phys. Chem. B. 109 (2005) 16161–16168. https://doi.org/10.1021/jp0441691.
- Z. Zhai, X. Yan, J. Xu, Z. Song, S. Shang, X. Rao, Phase Behavior and Aggregation in a Catanionic System Dominated by an Anionic Surfactant Containing a Large Rigid Group, Chem. – Eur. J. 24 (n.d.) 9033–9040. https://doi.org/10.1002/chem.201800628.
- P. Brown, C.P. Butts, J. Eastoe, Stimuli-responsive surfactants, Soft Matter. 9 (2013) 2365–2374. https://doi.org/10.1039/C3SM27716J.
- H. Sakai, K. Tsuchiya, K. Sakai, Stimuli-Responsible Viscoelastic Surfactant Solutions, in: Stimuli-Responsive Interfaces, Springer, Singapore, 2017: pp. 19–36. https://doi.org/10.1007/978-981-10-2463-4_3.
- B.W. Ninham, K. Larsson, P. Lo Nostro, Two sides of the coin. Part 1. Lipid and surfactant self-assembly revisited, Colloids Surf. B Biointerfaces. 152 (2017) 326–338. https://doi.org/10.1016/j.colsurfb.2017.01.022.
- B.W. Ninham, K. Larsson, P. Lo Nostro, Two sides of the coin. Part 2. Colloid and surface science meets real biointerfaces, Colloids Surf. B Biointerfaces. 159 (2017) 394–404. https://doi.org/10.1016/j.colsurfb.2017.07.090.
- B.W. Ninham, The Biological/Physical Sciences Divide, and the Age of Unreason, Substantia. 1 (2017) 7–24. https://doi.org/10.13128/Substantia-6.
- D. Tatini, M. Raudino, M. Ambrosi, E. Carretti, I. Davidovich, Y. Talmon, B.W. Ninham, P. Lo Nostro, Physicochemical characterization of green sodium oleate-based formulations. Part 1. Structure and rheology, J. Colloid Interface Sci. 590 (2021) 238–248. https://doi.org/10.1016/j.jcis.2021.01.040.
- Y. Lin, Y. Qiao, Y. Yan, J. Huang, Thermo-responsive viscoelastic wormlike micelle to elastic hydrogel transition in dual-component systems, Soft Matter. 5 (2009) 3047–3053. https://doi.org/10.1039/B906960G.
- P.L. Nostro, N. Stubicar, S.-H. Chen, Isotopic Effect in Phase Separation of Dioctanoylphosphatidylcholine/Water Micellar Solutions, Langmuir. 10 (1994) 1040–1043. https://doi.org/10.1021/la00016a015.
- T.M. Clausen, P.K. Vinson, J.R. Minter, H.T. Davis, Y. Talmon, W.G. Miller, Viscoelastic micellar solutions: microscopy and rheology, J. Phys. Chem. 96 (1992) 474–484. https://doi.org/10.1021/j100180a086.
- P.A. Hassan, S.R. Raghavan, E.W. Kaler, Microstructural Changes in SDS Micelles Induced by Hydrotropic Salt, Langmuir. 18 (2002) 2543–2548. https://doi.org/10.1021/la011435i.
- R. Byron Bird, P.J. Carreau, A nonlinear viscoelastic model for polymer solutions and melts—I, Chem. Eng. Sci. 23 (1968) 427–434. https://doi.org/10.1016/0009-2509(68)87018-6.
- R.H. Colby, Structure and linear viscoelasticity of flexible polymer solutions: comparison of polyelectrolyte and neutral polymer solutions, Rheol. Acta. 49 (2010) 425–442. https://doi.org/10.1007/s00397-009-0413-5.
- D. Tatini, D. Ciardi, C. Sofroniou, B.W. Ninham, P. Lo Nostro, Physicochemical characterization of green sodium oleate-based formulations. Part 2. Effect of anions, J. Colloid Interface Sci. 617 (2022) 399–408. https://doi.org/10.1016/j.jcis.2022.01.135.
- F. Sarri, D. Tatini, M. Raudino, M. Ambrosi, E. Carretti, P. Lo Nostro, Electro-Responsive Green Gels for Lower Environmental Impact Shale Gas Extraction, Energy Fuels. 33 (2019) 2057–2066. https://doi.org/10.1021/acs.energyfuels.8b04321.
- O.P. Artykulnyi, V.I. Petrenko, L.A. Bulavin, L. Almasy, N.A. Grigoryeva, M.V. Avdeev, V.L. Aksenov, On the Impact of Polyethylene Glycol on the Structure of Aqueous Micellar Solutions of Sodium Oleate According to Small-Angle Neutron Scattering, J. Surf. Investig. X-Ray Synchrotron Neutron Tech. 12 (2018) 1142–1148. https://doi.org/10.1134/S1027451018050543.
- D. Mijaljica, F. Spada, I.P. Harrison, Skin Cleansing without or with Compromise: Soaps and Syndets, Molecules. 27 (2022) 2010. https://doi.org/10.3390/molecules27062010.
- H. Bunjes, M.H.J. Koch, K. Westesen, Influence of emulsifiers on the crystallization of solid lipid nanoparticles, J. Pharm. Sci. 92 (2003) 1509–1520. https://doi.org/10.1002/jps.10413.
- M. Ratoi, H.A. Spikes, Lubricating Properties of Aqueous Surfactant Solutions, Tribol. Trans. 42 (1999) 479–486. https://doi.org/10.1080/10402009908982244.
- X.P. Li, L. Yu, Y.Q. Ji, B. Wu, G.Z. Li, L.Q. Zheng, New type flooding systems in enhanced oil recovery, Chin. Chem. Lett. 20 (2009) 1251–1254. https://doi.org/10.1016/j.cclet.2009.05.007.
- G.C. Kalur, S.R. Raghavan, Anionic Wormlike Micellar Fluids that Display Cloud Points: Rheology and Phase Behavior, J. Phys. Chem. B. 109 (2005) 8599–8604. https://doi.org/10.1021/jp044102d.
- F.E. Antunes, L. Coppola, D. Gaudio, I. Nicotera, C. Oliviero, Shear rheology and phase behaviour of sodium oleate/water mixtures, Colloids Surf. Physicochem. Eng. Asp. 297 (2007) 95–104. https://doi.org/10.1016/j.colsurfa.2006.10.030.
- Q. Cao, L. Yu, L.-Q. Zheng, G.-Z. Li, Y.-H. Ding, J.-H. Xiao, Rheological properties of wormlike micelles in sodium oleate solution induced by sodium ion, Colloids Surf. Physicochem. Eng. Asp. 312 (2008) 32–38. https://doi.org/10.1016/j.colsurfa.2007.06.024.
- P. Koshy, V.K. Aswal, M. Venkatesh, P.A. Hassan, Unusual Scaling in the Rheology of Branched Wormlike Micelles Formed by Cetyltrimethylammonium Bromide and Sodium Oleate, J. Phys. Chem. B. 115 (2011) 10817–10825. https://doi.org/10.1021/jp2053986.
- C. Flood, C.A. Dreiss, V. Croce, T. Cosgrove, G. Karlsson, Wormlike Micelles Mediated by Polyelectrolyte, Langmuir. 21 (2005) 7646–7652. https://doi.org/10.1021/la050326r.
- S. Khandavalli, J. Hendricks, C. Clasen, J.P. Rothstein, A comparison of linear and branched wormlike micelles using large amplitude oscillatory shear and orthogonal superposition rheology, J. Rheol. 60 (2016) 1331–1346. https://doi.org/10.1122/1.4965435.
- N.V. Thampi, R.P. John, K. Ojha, U.G. Nair, Effect of Salts, Alkali, and Temperature on the Properties of Sodium Oleate Hydrogel, Ind. Eng. Chem. Res. 55 (2016) 5805–5816. https://doi.org/10.1021/acs.iecr.6b00158.
- J. Huang, S. Zhang, Y. Feng, J. Li, H. Yan, F. He, G. Wang, Y. Liu, L. Wang, Rheological properties and application of wormlike micelles formed by sodium oleate/benzyltrimethyl ammonium bromide, Colloids Surf. Physicochem. Eng. Asp. 500 (2016) 222–229. https://doi.org/10.1016/j.colsurfa.2016.03.074.
- H. Lu, Q. Shi, Z. Huang, pH-Responsive Anionic Wormlike Micelle Based on Sodium Oleate Induced by NaCl, J. Phys. Chem. B. 118 (2014) 12511–12517. https://doi.org/10.1021/jp506809m.
- J. König, 2 - Food colour additives of synthetic origin, in: M.J. Scotter (Ed.), Colour Addit. Foods Beverages, Woodhead Publishing, Oxford, 2015: pp. 35–60. https://doi.org/10.1016/B978-1-78242-011-8.00002-7.
- H.M.D. Bandara, S.C. Burdette, Photoisomerization in different classes of azobenzene, Chem. Soc. Rev. 41 (2012) 1809–1825. https://doi.org/10.1039/C1CS15179G.
- R. D?browski, P. Kula, J. Herman, High Birefringence Liquid Crystals, Crystals. 3 (2013) 443–482. https://doi.org/10.3390/cryst3030443.
- D. Statman, I. Jánossy, Study of photoisomerization of azo dyes in liquid crystals, J. Chem. Phys. 118 (2003) 3222–3232. https://doi.org/10.1063/1.1538598.
- H. Chen, W. Chen, Y. Lin, Y. Xie, S.H. Liu, J. Yin, Visible and near-infrared light activated azo dyes, Chin. Chem. Lett. 32 (2021) 2359–2368. https://doi.org/10.1016/j.cclet.2021.03.020.
- H. Rau, Chapter 4 - Azo Compounds, in: H. Dürr, H. Bouas-Laurent (Eds.), Photochromism, Elsevier Science, Amsterdam, 2003: pp. 165–192. https://doi.org/10.1016/B978-044451322-9/50008-7.
- H. Oh, A.M. Ketner, R. Heymann, E. Kesselman, D. Danino, D.E. Falvey, S.R. Raghavan, A simple route to fluids with photo-switchable viscosities based on a reversible transition between vesicles and wormlike micelles, Soft Matter. 9 (2013) 5025–5033. https://doi.org/10.1039/C3SM00070B.
- Y. Lin, X. Cheng, Y. Qiao, C. Yu, Z. Li, Y. Yan, J. Huang, Creation of photo-modulated multi-state and multi-scale molecular assemblies via binary-state molecular switch, Soft Matter. 6 (2010) 902–908. https://doi.org/10.1039/B916721H.
- Y. Lu, T. Zhou, Q. Fan, J. Dong, X. Li, Light-responsive viscoelastic fluids based on anionic wormlike micelles, J. Colloid Interface Sci. 412 (2013) 107–111. https://doi.org/10.1016/j.jcis.2013.09.014.
- Y. Bi, H. Wei, Q. Hu, W. Xu, Y. Gong, L. Yu, Wormlike Micelles with Photoresponsive Viscoelastic Behavior Formed by Surface Active Ionic Liquid/Azobenzene Derivative Mixed Solution, Langmuir. 31 (2015) 3789–3798. https://doi.org/10.1021/acs.langmuir.5b00107.
- J. Jiang, G. Wang, Y. Ma, Z. Cui, B.P. Binks, Smart worm-like micelles responsive to CO2/N2 and light dual stimuli, Soft Matter. 13 (2017) 2727–2732. https://doi.org/10.1039/C7SM00185A.
- M. Salzano de Luna, V. Marturano, M. Manganelli, C. Santillo, V. Ambrogi, G. Filippone, P. Cerruti, Light-responsive and self-healing behavior of azobenzene-based supramolecular hydrogels, J. Colloid Interface Sci. 568 (2020) 16–24. https://doi.org/10.1016/j.jcis.2020.02.038.
- T. Liu, Q. Lei, J. Dong, X. Li, Y. Lu, T. Zhou, Light-responsive vesicles based on azobenzene containing imidazolium surfactants and sodium oleate, Colloid Polym. Sci. 297 (2019) 1489–1497. https://doi.org/10.1007/s00396-019-04557-8.
- D. Wang, R. Dong, P. Long, J. Hao, Photo-induced phase transition from multilamellar vesicles to wormlike micelles, Soft Matter. 7 (2011) 10713–10719. https://doi.org/10.1039/C1SM05949A.
- R. Kumar, S.R. Raghavan, Photogelling fluids based on light-activated growth of zwitterionic wormlike micelles, Soft Matter. 5 (2009) 797–803. https://doi.org/10.1039/B809452G.
- J. Li, M. Zhao, H. Zhou, H. Gao, L. Zheng, Photo-induced transformation of wormlike micelles to spherical micelles in aqueous solution, Soft Matter. 8 (2012) 7858–7864. https://doi.org/10.1039/C2SM25218J.
- H. Sakai, S. Taki, K. Tsuchiya, A. Matsumura, K. Sakai, M. Abe, Photochemical Control of Viscosity Using Sodium Cinnamate as a Photoswitchable Molecule, Chem. Lett. 41 (2012) 247–248. https://doi.org/10.1246/cl.2012.247.
- M. Zhao, M. Gao, C. Dai, C. Zou, Z. Yang, X. Wu, Y. Liu, Y. Wu, S. Fang, W. Lv, Investigation of Novel Triple-Responsive Wormlike Micelles, Langmuir. 33 (2017) 4319–4327. https://doi.org/10.1021/acs.langmuir.7b01011.
- E. Nabi, M. Drechsler, M. Gradzielski, Phase behaviour and vesicle formation in catanionic mixtures of Na oleate and alkyl trimethyl ammonium bromide and its salt-free version, Colloid Polym. Sci. 293 (2015) 3119–3130. https://doi.org/10.1007/s00396-015-3737-y.
- D. Tatini, F. Sarri, P. Maltoni, M. Ambrosi, E. Carretti, B.W. Ninham, P. Lo Nostro, Specific ion effects in polysaccharide dispersions, Carbohydr. Polym. 173 (2017) 344–352. https://doi.org/10.1016/j.carbpol.2017.05.078.
- D. Tatini, P. Tempesti, F. Ridi, E. Fratini, M. Bonini, P. Baglioni, Pluronic/gelatin composites for controlled release of actives, Colloids Surf. B Biointerfaces. 135 (2015) 400–407. https://doi.org/10.1016/j.colsurfb.2015.08.002.
- B.A. Schubert, E.W. Kaler, N.J. Wagner, The Microstructure and Rheology of Mixed Cationic/Anionic Wormlike Micelles, Langmuir. 19 (2003) 4079–4089. https://doi.org/10.1021/la020821c.
- M.M. Cross, Rheology of non-Newtonian fluids: A new flow equation for pseudoplastic systems, J. Colloid Sci. 20 (1965) 417–437. https://doi.org/10.1016/0095-8522(65)90022-X.
- Toshiyuki. Shikata, Hirotaka. Hirata, Tadao. Kotaka, Micelle formation of detergent molecules in aqueous media: viscoelastic properties of aqueous cetyltrimethylammonium bromide solutions, Langmuir. 3 (1987) 1081–1086. https://doi.org/10.1021/la00078a035.
- T. Shikata, H. Hirata, T. Kotaka, Micelle formation of detergent molecules in aqueous media. 2. Role of free salicylate ions on viscoelastic properties of aqueous cetyltrimethylammonium bromide-sodium salicylate solutions, Langmuir. 4 (1988) 354–359. https://doi.org/10.1021/la00080a019.
- C. L Barrie, P.C. Griffiths, R.J. Abbott, I. Grillo, E. Kudryashov, C. Smyth, Rheology of aqueous carbon black dispersions, J. Colloid Interface Sci. 272 (2004) 210–217. https://doi.org/10.1016/j.jcis.2003.12.004.
- T. Sochi, Non-Newtonian flow in porous media, Polymer. 51 (2010) 5007–5023. https://doi.org/10.1016/j.polymer.2010.07.047.
- G. Polacco, S. Filippi, F. Merusi, G. Stastna, A review of the fundamentals of polymer-modified asphalts: Asphalt/polymer interactions and principles of compatibility, Adv. Colloid Interface Sci. 224 (2015) 72–112. https://doi.org/10.1016/j.cis.2015.07.010.
- M.F. Nazar, S. Murtaza, B. Ijaz, M. Asfaq, M.A. Mohsin, Photophysical Investigations of Carmoisine Interacting with Conventional Cationic Surfactants Under Different pH Conditions, J. Dispers. Sci. Technol. 36 (2015) 18–27. https://doi.org/10.1080/01932691.2014.884465.
- A. Basu, G. Suresh Kumar, Minor Groove Binding of the Food Colorant Carmoisine to DNA: Spectroscopic and Calorimetric Characterization Studies, J. Agric. Food Chem. 62 (2014) 317–326. https://doi.org/10.1021/jf404960n.
- S.J. Candau, E. Hirsch, R. Zana, Light scattering investigations of the behavior of semidilute aqueous micellar solutions of cetyltrimethylammonium bromide: Analogy with semidilute polymer solutions, J. Colloid Interface Sci. 105 (1985) 521–528. https://doi.org/10.1016/0021-9797(85)90327-3.
- L.J. Magid, Z. Han, Z. Li, P.D. Butler, Tuning Microstructure of Cationic Micelles on Multiple Length Scales: The Role of Electrostatics and Specific Ion Binding, Langmuir. 16 (2000) 149–156. https://doi.org/10.1021/la990686c.
- R.D. Koehler, S.R. Raghavan, E.W. Kaler, Microstructure and Dynamics of Wormlike Micellar Solutions Formed by Mixing Cationic and Anionic Surfactants, J. Phys. Chem. B. 104 (2000) 11035–11044. https://doi.org/10.1021/jp0018899.
- H. Hoffmann, Viscoelastic Surfactant Solutions, in: Struct. Flow Surfactant Solut., American Chemical Society, 1994: pp. 2–31. https://doi.org/10.1021/bk-1994-0578.ch001.
- M. In, G.G. Warr, R. Zana, Dynamics of Branched Threadlike Micelles, Phys. Rev. Lett. 83 (1999) 2278–2281. https://doi.org/10.1103/PhysRevLett.83.2278.
- A. Parker, W. Fieber, Viscoelasticity of anionic wormlike micelles: effects of ionic strength and small hydrophobic molecules, Soft Matter. 9 (2012) 1203–1213. https://doi.org/10.1039/C2SM27078A.
- C. Rodriguez-Abreu, K. Aramaki, Y. Tanaka, M.A. Lopez-Quintela, M. Ishitobi, H. Kunieda, Wormlike micelles and microemulsions in aqueous mixtures of sucrose esters and nonionic cosurfactants., J. Colloid Interface Sci. 291 (2005) 560–569. https://doi.org/10.1016/j.jcis.2005.05.018.
- N. Naito, D.P. Acharya, J. Tanimura, H. Kunieda, Rheological Behavior of Wormlike Micellar Solutions in Mixed Nonionic Systems of Polyoxyethylene Phytosterol-Polyoxyethylene Dodecyl Ether, J. Oleo Sci. 53 (2004) 599–606. https://doi.org/10.5650/jos.53.599.
- R.G. Shrestha, C. Rodriguez-Abreu, K. Aramaki, Wormlike Micelles in Mixed Amino Acid Surfactant/Nonionic Surfactant Aqueous Systems and the Effect of Added Electrolytes, J. Oleo Sci. 58 (2009) 243–254. https://doi.org/10.5650/jos.58.243.
- H. Rehage, H. Hoffmann, Viscoelastic surfactant solutions: model systems for rheological research, Mol. Phys. 74 (1991) 933–973. https://doi.org/10.1080/00268979100102721.
- S.J. Candau, R. Oda, Linear viscoelasticity of salt-free wormlike micellar solutions, Colloids Surf. Physicochem. Eng. Asp. 183–185 (2001) 5–14. https://doi.org/10.1016/S0927-7757(01)00535-0.
- S.A. Rogers, M.A. Calabrese, N.J. Wagner, Rheology of branched wormlike micelles, Curr. Opin. Colloid Interface Sci. 19 (2014) 530–535. https://doi.org/10.1016/j.cocis.2014.10.006.
- M. Buchanan, M. Atakhorrami, J.F. Palierne, F.C. MacKintosh, C.F. Schmidt, High-frequency microrheology of wormlike micelles, Phys. Rev. E. 72 (2005) 011504. https://doi.org/10.1103/PhysRevE.72.011504.
- J. Honerkamp, J. Weese, A nonlinear regularization method for the calculation of relaxation spectra, Rheol. Acta. 32 (1993) 65–73. https://doi.org/10.1007/BF00396678.
- E. Carretti, C. Matarrese, E. Fratini, P. Baglioni, L. Dei, Physicochemical characterization of partially hydrolyzed poly(vinyl acetate)–borate aqueous dispersions, Soft Matter. 10 (2014) 4443–4450. https://doi.org/10.1039/C4SM00355A.
- N. Garti, A. Aserin, S. Ezrahi, I. Tiunova, G. Berkovic, Water Behavior in Nonionic Surfactant Systems I: Subzero Temperature Behavior of Water in Nonionic Microemulsions Studied by DSC, J. Colloid Interface Sci. 178 (1996) 60–68. https://doi.org/10.1006/jcis.1996.0093.
- M. Ambrosi, P.L. Nostro, L. Fratoni, L. Dei, B.W. Ninham, S. Palma, R.H. Manzo, D. Allemandi, P. Baglioni, Water of hydration in coagels, Phys. Chem. Chem. Phys. 6 (2004) 1401–1407. https://doi.org/10.1039/B314061J.
- P.C. Schulz, DSC analysis of the state of water in surfactant-based microstructures, J. Therm. Anal. Calorim. 51 (1998) 135–149. https://doi.org/10.1007/BF02719017.
- M. Fernandez-Tarrio, C. Alvarez-Lorenzo, A. Concheiro, Calorimetric approach to tetronic/water interactions, J. Therm. Anal. Calorim. 87 (2007) 171–178. https://doi.org/10.1007/s10973-006-7834-5.
- P.C. Schulz, J.E. Puig, The state of water in lyotropic liquid crystals, Colloids Surf. Physicochem. Eng. Asp. 71 (1993) 83–90. https://doi.org/10.1016/0927-7757(93)80030-I.
- N. Garti, Thermal Behavior of Dispersed Systems, CRC Press, 2000.
- K. Nakamura, T. Hatakeyama, H. Hatakeyama, Relationship between hydrogen bonding and bound water in polyhydroxystyrene derivatives, Polymer. 24 (1983) 871–876. https://doi.org/10.1016/0032-3861(83)90206-9.
- D. Senatra, G. Gabrielli, G.G.T. Guarini, Experimental Evidence of Interphasal Water in Water-in-Oil Microemulsions, EPL Europhys. Lett. 2 (1986) 455. https://doi.org/10.1209/0295-5075/2/6/008.
- Chaplin, Martin, Polysaccharide hydration, (n.d.). http://www1.lsbu.ac.uk/water/polysaccharide_hydration.html (accessed September 27, 2016).
- L. Guan, H. Xu, D. Huang, hatak, J. Polym. Res. 18 (2010) 681–689. https://doi.org/10.1007/s10965-010-9464-7.
- T. Hatakeyama, M. Tanaka, H. Hatakeyama, Thermal Properties of Freezing Bound Water Restrained by Polysaccharides, J. Biomater. Sci. Polym. Ed. 21 (2010) 1865–1875. https://doi.org/10.1163/092050610X486946.
- N. Garti, Thermal Behavior of Dispersed Systems, CRC Press, 2000.