Singlet Dioxygen ¹O?, its Generation, Physico-Chemical Properties and its Possible Hormetic Behavior in Cancer Therapy
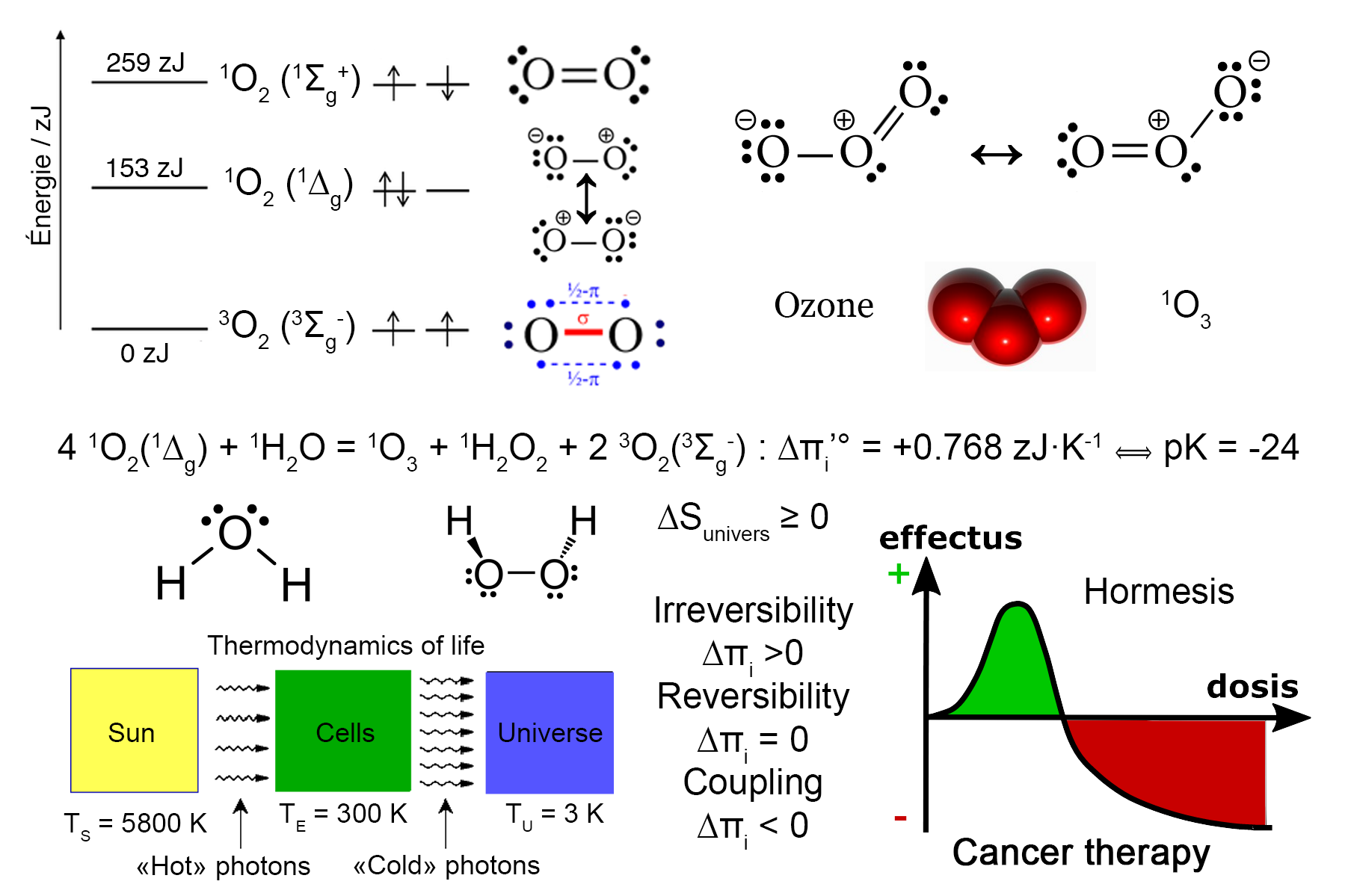
Published 2022-03-07
Keywords
- cancer,
- phototherapy,
- Warburg’s effect,
- radiation therapy,
- singlet oxygen
- chemotherapy,
- oxygen effect ...More
How to Cite
Copyright (c) 2022 Marc Henry, Miro Radman, Luc Benichou, Khalid O. Alfarouk, Laurent Schwartz

This work is licensed under a Creative Commons Attribution 4.0 International License.
Abstract
Singlet dioxygen 1O2 is one excited state among the three other possible spectroscopic states of molecular oxygen. Here, we first describe the use of published spectroscopic data and thermodynamic modeling based on irreversible entropy production. Such concepts are further applied to the synthesis of singlet dioxygen and its reactions with crucial biological molecules. In a last section, we suggest that singlet dioxygen and ozone may be responsible for the success of radiation therapy, that has been used to treat cancer successfully for over 120 years. Its precise mechanism of action remains controversial. We thus aim to clarify the role of singlet oxygen in radiotherapy and chemotherapy. A partial conversion of ionizing radiation in the body into thermal photons could be assumed. The antitumor effect may involve these thermal photons, such as the one delivered by red/infrared sources. Thermal photons (wavelengths of 635 nm and 1270 nm) convert triplet dioxygen into singlet dioxygen by changing the spin of its outer electrons. Despite its short half-life, Singlet dioxygen is responsible for the activation of multiple free radicals (such as hydrogen peroxide), which may target proteins and DNA, induce either apoptosis or oxidative phosphorylation. At moderate concentrations, thermodynamic data suggests that singlet dioxygen may readily react with water to form a potent pro-apoptotic molecule (ozone), thus decreasing cancer growth. However, at high concentration cytotoxic effects against all kind of cells occurs. This strongly suggests a non-linear hormetic behavior of singlet dioxygen. It is also proposed that cytotoxic chemotherapy induces the same free radicals that singlet dioxygen does. There are also other ways to enhance the production of singlet dioxygen, such as phototherapy using Methylene Blue for instance. As a source of reactive oxygen species (ROS), singlet oxygen could thus be a common agent active both in radiotherapy and chemotherapy. It is probable that the activity of radiation therapy and chemotherapy may be mediated by the conversion of triplet to singlet oxygen. This may explain the oxygen effect such as described in radiotherapy and chemotherapy.
References
- Feeund L. Elements of General Radiotherapy for Practitioners. Arch. Roentgen Ray. 1904;9:33–34.
- Calabrese EJ, Calabrese V. Low dose radiation therapy (LD-RT) is effective in the treatment of arthritis: Animal model findings. Int. J. Radiat. Biol. 2013;89:287–294.
- Prise KM, Pinto M, Newman HC, et al. A review of studies of ionizing radiation-induced double-strand break clustering. In: Radiation Research.Vol 156. Radiation Research Society; 2001:572–576.
- Rieger KE, Hong WJ, Tusher VG, et al. Toxicity from radiation therapy associated with abnormal transcriptional responses to DNA damage. Proc. Natl. Acad. Sci. U. S. A. 2004;101:6635–6640.
- Wu AHB, Feng YJ, Moore R, et al. Characterization of cardiac-troponin subunit release into serum after acute myocardial infarction and comparison of assays for troponin T and I. Clin. Chem. 1998;44:1198–1208.
- Sahani D V., Kalva SP, Hamberg LM, et al. Assessing tumor perfusion and treatment response in rectal cancer with multisection CT: Initial observations. Radiology. 2005;234:785–792.
- Radman M. Protein damage, radiation sensitivity and aging. DNA Repair (Amst). 2016;44:186–192.
- Sies H. (editor), "Oxidative Stress, 1st Edition : Eustress and Distress", Academic Press (2019).
- Henry M, Schwartz L. Entropy export as the driving force of evolution. Substantia. 2019;3(2) suppl. 3:29–56.
- Schwartz L, Devin A, Bouillaud F, et al. Entropy as the Driving Force of Pathogenesis: an Attempt of Diseases Classification Based on the Laws of Physics. Substantia. 2020; 4(2): 7-13.
- Henry M. Thermodynamics of Life. Substantia. 2021; 5(1): 43-71.
- Mattson M. P., Hormesis defined, Ageing Res Rev. (2008); 7(1): 1–7.
- Warburg O. On the origin of cancer cells. Science. 1956;123:309–14.
- Schwartz L, Supuran CT, Alfarouk KO. The Warburg effect and the Hallmarks of Cancer. Anticancer. Agents Med. Chem. 2017;17:164–170.
- Wen H, Ting JPY, O’Neill LAJ. A role for the NLRP3 inflammasome in metabolic diseases - Did Warburg miss inflammation? Nat. Immunol. 2012;13:352–357.
- Schwartz L, Peres S, Jolicoeur M, et al. Cancer and Alzheimer’s disease: intracellular pH scales the metabolic disorders. Biogerontology. 2020.
- Hamblin MR, Nelson ST, Strahan JR. Photobiomodulation and Cancer: What Is the Truth? Photomed. Laser Surg. 2018;36:241–245.
- Kajagar BM, Godhi AS, Pandit A, et al. Efficacy of Low Level Laser Therapy on Wound Healing in Patients with Chronic Diabetic Foot Ulcers-A Randomised Control Trial. Indian J. Surg. 2012;74:359–363.
- De Oliveira Chami V, Maracci LM, Tomazoni F, et al. Rapid LLLT protocol for myofascial pain and mouth opening limitation treatment in the clinical practice: An RCT. Cranio - J. Craniomandib. Pract. 2020.
- Silveira PCL, Scheffer DDL, Glaser V, et al. Low-level laser therapy attenuates the acute inflammatory response induced by muscle traumatic injury. Free Radic. Res. 2016;50:503–513.
- Tezcan S, Ozturk FU, Uslu N, et al. Carpal tunnel syndrome: Evaluation of the effects of low-level laser therapy with ultrasound strain imaging. J. Ultrasound Med. 2019;38:113–122.
- Poiani G da CR, Zaninotto AL, Carneiro AMC, et al. Photobiomodulation using low-level laser therapy (LLLT) for patients with chronic traumatic brain injury: A randomized controlled trial study protocol. Trials. 2018;19.
- Schiffer F, Johnston AL, Ravichandran C, et al. Psychological benefits 2 and 4 weeks after a single treatment with near infrared light to the forehead: a pilot study of 10 patients with major depression and anxiety. Behav. Brain Funct. 2009;5:46.
- Salehpour F, Gholipour-Khalili S, Farajdokht F, et al. Therapeutic potential of intranasal photobiomodulation therapy for neurological and neuropsychiatric disorders: A narrative review. Rev. Neurosci. 2020;31:269–286.
- Merry GF, Munk MR, Dotson RS, et al. Photobiomodulation reduces drusen volume and improves visual acuity and contrast sensitivity in dry age-related macular degeneration. Acta Ophthalmol. 2017;95:e270–e277.
- Lewis GN. The atom and the molecule. J. Am. Chem. Soc. 1916;38:762–785.
- Langmuir I. Types of valence. Science (80-. ). 1921;54:59–67.
- Reed J. L. The lewis structure: An expanded perspective. J. Chem. Educ. 1994;71:98–100.
- Allen RC. Role of oxygen in phagocyte microbicidal action. In: Environmental Health Perspectives.Vol 102. Public Health Services, US Dept of Health and Human Services; 1994:201–208.
- Devasagayam TPA, Kamat JP. Biological significance of singlet oxygen. In: Indian Journal of Experimental Biology.Vol 40.; 2002:680–692.
- O’Reilly DE, Peterson EM. Rotational correlation times and coefficients of viscosity of electrolytic solutions. J. Phys. Chem. 1970;74:3280–3285.
- Alberty RA. Thermodynamics of Biochemical Reactions. Hoboken, NJ, USA: John Wiley & Sons, Inc.; 2003.
- Krieger-Liszkay A. Singlet oxygen production in photosynthesis. In: Journal of Experimental Botany.Vol 56. J Exp Bot; 2005:337–346.
- Laloi C, Havaux M. Key players of singlet oxygen-induced cell death in plants. Front. Plant Sci. 2015;6.
- Patel A, Malinovska L, Saha S, et al. ATP as a biological hydrotrope. Science (80-. ). 2017;356:753–756.
- Agledal L, Niere M, Ziegler M. The phosphate makes a difference: Cellular functions of NADP. Redox Rep. 2010;15:2–10.
- Henry M., « The topological and quantum structure of zoemorphic water », in Aqua Incognita: Why Ice Floats on Water and Galileo 400 Years on, P. Lo Nostro & B. W. Ninham Eds, Connor Court Pub., Ballarat (2014), chap IX, 197-239.
- Liang X, Wang KK, Zhu TC. Singlet oxygen dosimetry modeling for photodynamic therapy. In: Kessel DH, Hasan T, eds. Optical Methods for Tumor Treatment and Detection: Mechanisms and Techniques in Photodynamic Therapy XXI.Vol 8210. SPIE; 2012:82100T.
- Richardson RB, Harper ME. Mitochondrial stress controls the radiosensitivity of the oxygen effect: Implications for radiotherapy. Oncotarget. 2016;7:21469–21483.
- Berneburg M, Grether-Beck S, Kürten V, et al. Singlet oxygen mediates the UVA-induced generation of the photoaging- associated mitochondrial common deletion. J. Biol. Chem. 1999;274:15345–15349.
- Wentworth Jr. P, Wentworth AD, Zhu Xueyong, Wilson IA, Janda KD, Eschenmoser A,
- Lerner RA. Evidence for the production of trioxygen species during antibody-catalyzed chemical modification of antigens. Proc. Natl. Acad Sci. USA, 2003; 100: 1490-1493.
- Beall PT, Hazlewood CF, Rao PN. Nuclear magnetic resonance patterns of intracellular water as a function of HeLa cell cycle. Science (80-. ). 1976;192:904–907.
- Beall PT, Brinkley BR, Chang DC, et al. Microtubule Complexes Correlated with Growth Relaxation Times in Human Breast Cancer Cells. Cancer Res. 1982;42.
- Gniadecka M, Nielsen OF, Wulf HC. Water content and structure in malignant and benign skin tumours. J. Mol. Struct. 2003;661–662:405–410.
- McIntyre GI. Cell hydration as the primary factor in carcinogenesis: A unifying concept. Med. Hypotheses. 2006;66:518–526.
- Davidson R, Lauritzen A, Seneff S. Biological Water Dynamics and Entropy: A Biophysical Origin of Cancer and Other Diseases. Entropy. 2013;15:3822–3876.
- Arslan H, Dolu?an YB, Ay AN. Measurement of the Penetration Depth in Biological Tissue for Different Optical Powers. 2017.
- Conklin KA. Chemotherapy-associated oxidative stress: Impact on chemotherapeutic effectiveness. Integr. Cancer Ther. 2004;3:294–300.
- Tardivo JP, Del Giglio A, De Oliveira CS, et al. Methylene blue in photodynamic therapy: From basic mechanisms to clinical applications. Photodiagnosis Photodyn. Ther. 2005;2:175–191.